Many of us at some point in our lives have pondered that rather esoteric question of what the world must have been like at the very beginning of existence. Michael Strickland, Ph.D., professor and chair of Kent State University’s Department of Physics, actually knows.
In March, Strickland received a continuation grant of $230,000 from the U.S. Department of Energy for his research into “Non-equilibrium Dynamics of the Quark Gluon Plasma.” Using this funding, Strickland and his team have developed the world’s leading approach to describe non-equilibrium evolution of highly relativistic systems.
What does that mean exactly?
To put it more plainly, the research that Strickland and his team have been able to conduct essentially recreates the state of the universe up to approximately 1 microsecond after the Big Bang, increasing our understanding of the behavior of matter under extreme conditions.
As it turns out though, right after the Big Bang, matter as we know it didn’t exist yet.
“Atoms had not yet formed. Atomic nuclei had not yet formed. Even the protons and neutrons that make up the atomic nuclei had not yet formed,” said Strickland. “Instead, the entire universe was a primordial form of matter called a quark-gluon plasma (QGP).”
As Strickland explains it, quarks and gluons are the fundamental particles that make up protons, neutrons and all other “hadrons,” which is the class name for particles made up of quarks and gluons. The fact that the early universe was a quark-gluon plasma is due to the fact it was extremely hot, he said.
“If the temperature exceeds approximately 1012 Kelvin, then the QGP is the preferred phase of matter. Much like at high temperature, the preferred phase of H20 is a gas instead of a liquid,” Strickland explained.
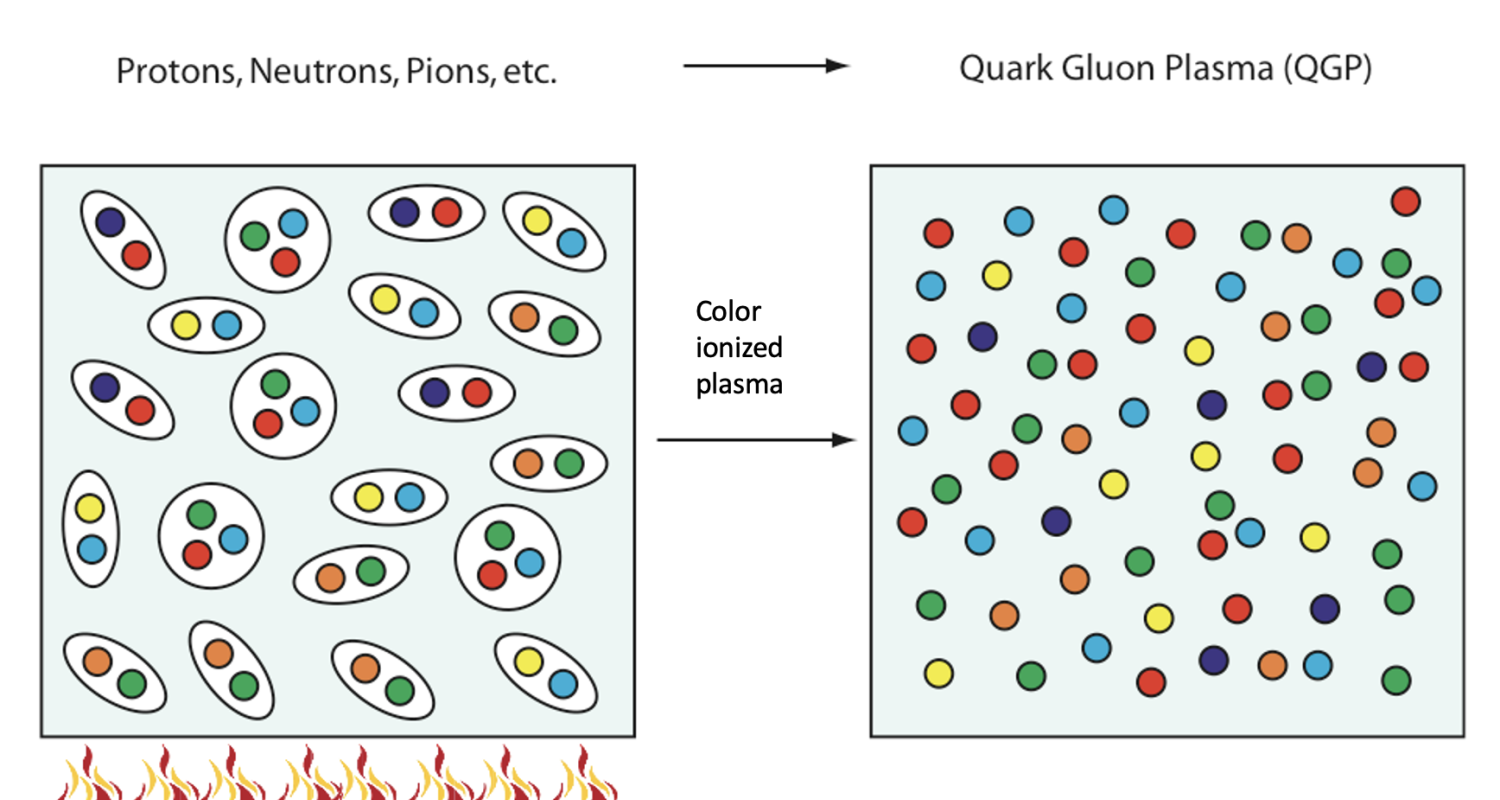
Strickland said that the existence of a phase transition between quark-gluon plasma and hadronic matter (protons, neutrons, etc.) was predicted in the late 1970s. (The theory, known as Quantum Chromodynamics, won the Nobel Prize in 2004.) Since those early predictions, particle collision experiments have been carried out at the Relativistic Heavy Ion Collider at the Brookhaven National Laboratory on Long Island, New York, as well as at the Large Hadron Collider at CERN in Europe. The experiments essentially replicate a scenario similar to that around the time of the Big Bang and basically “melt” nuclear matter with nuclear collisions at speeds close to the speed of light. These experiments have enabled researchers to determine critical information about the early universe.
This type of cutting-edge work illustrates Kent State’s national recognition as a top tier research institution. The research that the Department of Energy grant supports has been continually funded since 2015 and has brought in over $1.5 million in external funding. According to Strickland, the funding has been used to train graduate students and hire postdoctoral students to work on the project, helping to prepare today’s and tomorrow’s workforce.
“It has supported the Ph.D.s of approximately 10 Ph.D. students and the work of four postdocs,” he said.
The project is up for a renewal of the continuation grant again in early 2024.
Learn more about the Department of Physics here.